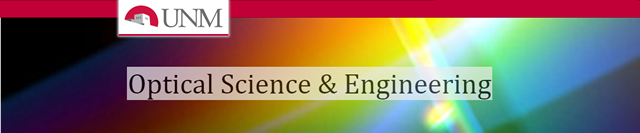
Optical Science and Engineering ETDs
Publication Date
Spring 5-10-2019
Abstract
Future smart-lighting systems are expected to deliver adaptively color-tunable and high-quality lighting that is energy efficient while also offering integrated visible-light wireless communication services. To enable these systems at a commercial level, inexpensive and fast sensors with spectral-sensing capability are required. CMOS-compatible silicon avalanche photodiodes (APDs) can be an excellent fit to this problem due to their excellent sensitivity, high speeds and cost effectiveness; however, color sensing is a challenge without resorting to expensive spectral filters, as done in commercially. To address this challenge, we have recently designed and modeled a novel CMOS-compatible dual-junction APD. The device outputs two photocurrents simultaneously, one for each junction, and each junction is controlled independently via a bias voltage so that each photocurrent can exhibit its own avalanche amplification factors and sensitivity. What is unique here is that each APD responds differently to different wavelengths because of (1) the wavelength-dependent nature of the light-absorption profile in the device, and (2) the dependence of the avalanche multiplication process on the location of photon absorptions (and hence on wavelength). The idea is to produce a series of photocurrent pairs, at judiciously prescribed pairs of biases for each acquisition, which would contain sufficient spectral information about the light as well as its intensity, which can be extracted from the data via an algorithm. Modeling shows that we can ideally use a pair of biases to detect the color and intensity within 10 nm spectral resolution in the 440-650 nm wavelength range using a maximum likelihood (ML) algorithm. In practice, however, the spectrum and intensity must be calculated from the series of measured photocurrent pairs using a ML algorithm, which would employ the wavelength and bias-voltage dependent joint probability density function (pdf) of the two photocurrents. The pdf accounts for both APDs’ responsivities in the presence of Johnson (electronic amplifier) noise, dark current, and most importantly, the avalanche gain uncertainty, represented by the excess-noise factor at each wavelength.
We have designed a programmable, inexpensive (CMOS compatible) dual-junction silicon APD that outputs the intensity and spectrum of the sensed illumination that addresses the needs of smart lighting without the use of any spectral filters. The efforts include: (1) the computation of the mean gain, excess noise factor and avalanche breakdown voltage for the dual APD as a function of bias and wavelength, (2) development of an exact analytical formula for excess noise factor under mixed injection, and (3) development of an exhaustive computation of the ML estimates of the intensity and spectral profile. The ML computations include an exhaustive search of a multidimensional space of wavelength and intensity values.
As mentioned earlier, each APD responds differently to different wavelengths due to the dependence of the avalanche multiplication process on the location of photon absorptions. This phenomenon leads to the mixed-injection avalanche multiplication process and the mean gain uncertainty, i.e., excess noise factor. Note that the well-known analytical formula for the excess noise factor associated with avalanche photodiodes (APDs), developed by R. J. McIntyre in 1966, assumes the injection of either an electron or a hole at the edge of the APD’s avalanche region. This formula, however, is not applicable in cases when photons are absorbed inside the avalanche region, and its use may severely underestimate or overestimate the actual excess noise factor depending on the absorption profile and the hole-to-electron ionization coefficient ratio, k. Here, an easy-to-use exact analytical formula is derived for excess noise factor while taking into account a mixed-carrier initiated avalanche multiplication process, which is triggered by a parent electron-hole pair at an arbitrarily specified location within the multiplication region. In addition, an expression for the excess noise factor is presented in the case when the location of the parent electron-hole pair within the multiplication region obeys an arbitrary exponential distribution. The results show that in contrast to the case of edge parent-electron injection, when mixed injection is allowed even a small level of hole ionization (e.g., small k~0.0001) causes the excess noise factor to increase dramatic, depending on the absorption profile as it ranges from narrow to flat within the multiplication region. The theoretical results are validated against experimental results for Si APDs.
Degree Name
Optical Science and Engineering
Level of Degree
Doctoral
Department Name
Optical Science and Engineering
First Committee Member (Chair)
Daniel Feezell
Second Committee Member
Majeed M. Hayat
Third Committee Member
Payman Zarkesh-Ha
Fourth Committee Member
Mansoor Sheik-Bahae
Keywords
Spectral sensor, CMOS APDs, Mixed injection, Maximum likelihood estimator, Smart lighting, Visible light communication
Sponsors
National Science Foundation and Sandia National Laboratories
Document Type
Dissertation
Language
English
Recommended Citation
Hossain, Md Mottaleb. "Algorithmic Multi-Color CMOS Avalanche Photodiodes for Smart-Lighting Applications." (2019). https://digitalrepository.unm.edu/ose_etds/71
Included in
Electromagnetics and Photonics Commons, Electronic Devices and Semiconductor Manufacturing Commons, Semiconductor and Optical Materials Commons