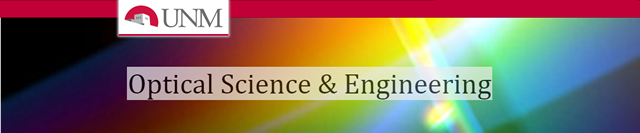
Optical Science and Engineering ETDs
Publication Date
Spring 7-17-2017
Abstract
For applications requiring the detection of low light levels, avalanche photodiodes (APDs) are the preferred detector of choice. The reason for this is their internal gain, which improves the APDs sensitivity (up to 5 − 10 dB) when compared to PIN-based photodiodes. The internal gain for an APD comes from the process of impact ionization process; however, due to its stochastic nature, the multiplication gain comes at the expense of extra noise. This multiplication noise, called the excess noise, is a measure of the gain uncertainty. Despite significant progress made in the fabrication process for the APDs, the excess noise remains a challenge for state-of- the-art APDs and limits their performance measures such as signal-to-noise ratio (SNR). Although there is present a formulation of noise based on the local-theory model, it fails to provide accurate predictions for thin (< 1μ m) devices. This is due to the presence of dead space, the distance a carrier must travel before it can impact ionize, which reduces the uncertainty of the stochastic process and the excess noise. Additionally, the buildup time, defined as the time required for all the impact ionizations to complete, increases as the mean gain increases. This leads to the presence of intersymbol interference within the device, which is detrimental to the device performance, especially at higher speeds. There is, therefore, a need to characterize the perfomance measures such as the mean gain and the noise in the presence of dead space for thin devices.
Presented in this work are modeling techniques which address these issues. A look is taken at 3D device structures for which the field characteristics as well as the material ionization coefficients vary along the multiplication path; this is the practical culmination of the dead space multiplication theory (DSMT). While the linear mode analysis using DSMT for 3D structures has recently been implemented, we show here the adapted 3D DSMT model for transient and Geiger modes analysis, including the evaluation of the gain-bandwidth product and the photon detection efficiency. A sample 3D Si-Ge lateral APD is used to develop and test this model.
Even for 1D APDs, implementation of the DSMT is cumbersome. In an effort to simplify it, a look is taken at the scenario when the ionization coefficients for holes and electrons in the APD multiplier may be taken to be equal. This may be the case when the multiplication width is small and the electric field is high. It is shown, mathematically, that by doing so, the DSMT is simplified considerably. The APD devices are then modeled for three different materials for which this assumption may be true. This provides us practical limits for which the formulations may be used.
Another instance in which the DSMT implementation is simplified by assuming that the ionization coefficients are completely disparate. Such is the case in InAs, which has recently been shown to be an electron-only ionization material. For this case, it is verified that the behavior of the APD deviates from that predicted by McIntyre’s local theory and that the noise in such APDs is reduced. In addition, earlier predictions made by Hayat et al. for mean gain using the asymptotic formulas are tested against the results from the recursive integral equations modeling data. The use of such material in IR detection, which has many applications in remote sensing, medical imaging, and environmental monitoring, is also discussed in the context of a novel, low-noise, high-gain, design APDs with a heterojunction design.
This dissertation, therefore, extends the capability in modeling the performance of APDs for different configurations. In the presence of the dead space, the characterization of practical 3D devices using the DSMT as well as the simplification in the gain and noise calculation for 1D devices provide an invaluable tool in under- standing the behavior of thin APDs. In addition, the modeling of a new design of heterojunction APDs for mid-IR detection allows for a new breed of designs to be developed with lower customizable noise and improved gain characteristics.
Degree Name
Optical Science and Engineering
Level of Degree
Doctoral
Department Name
Optical Science and Engineering
First Committee Member (Chair)
Prof. Majeed Hayat
Second Committee Member
Prof. Daniel Feezell
Third Committee Member
Prof. Payman Zarkesh-Ha
Fourth Committee Member
Prof. Francisco Becerra Chavez
Fifth Committee Member
Dr. Paul Davids
Keywords
photodetectors photon spad avalanche photodiodes
Document Type
Dissertation
Language
English
Recommended Citation
Jamil, Erum. "Analytical modeling and characterization for avalanche photodiodes." (2017). https://digitalrepository.unm.edu/ose_etds/58