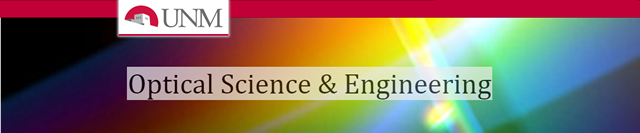
Optical Science and Engineering ETDs
Publication Date
2-9-2011
Abstract
In this dissertation we applied methods of ultrafast terahertz (THz) spectroscopy to study several aspects of semiconductor physics and in particular of collective mode excitations in semiconductors. We detect and analyze THz radiation emitted by these collective modes to reveal the underlying physics of many-body interactions. We review a design, implementation and characterization of our ultrafast terahertz (THz) time-domain spectroscopy setup, with additional features of mid-infrared tunability and coherent as well as incoherent detection capabilities. Temperature characterization of the collective plasmon excitation in indium antimonide (InSb) is presented to reveal the importance of non-parabolicity corrections in quantitative description. We also obtain electronic mobility from the radiation signals, which, once corrected for ultrafast scattering mechanisms, is in good agreement with DC Hall mobility measurements. Exhibited sensitivity to non-parabolicity and electronic mobility is applicable to non-contact characterization of electronic transport in nanostructures. As a first goal of this work, we have addressed the possibility of an all-optical control of the electronic properties of condensed matter systems on an ultrafast time scale. Using femtosecond pulses we have demonstrated an ability to impose a nearly 20% blue-shift of the plasma frequency in InSb. Preliminary investigations of control of the electron dynamics using third-order nonlinearity were also carried out in solid state and gaseous media. In particular, we have experimentally verified the THz coherent control in air-breakdown plasmas and have demonstrated the ability to induce quantum-interference current control in indium arsenide crystals. As a second focus of this dissertation, we have addressed manipulation of the plasmon modes in condensed matter systems. After development of the analytical model of radiation from spatially extended longitudinal modes, we have applied it to analysis of two experiments. In first, we established the ability to control plasmon modes in InSb by means of a plasmonic one dimensional cavity. By control of the cavity geometry, we shifted the plasmon mode into the regime where non-local electron-electron interaction is enforced. We observed the consequential Landau damping of the collective mode, in good agreement with the predictions made within the random-phase approximation. In the second experiment we have invoked plasmon confinement in all three dimensions via a nanowire geometry. We observed enhancement of terahertz emission which we attributed to leaky modes of the waveguide. We attributed this emission to the low-energy acoustic surface plasmon mode of the nanowire, which was also supported by our numerical modeling results and independent DC electronic measurements.
Degree Name
Optical Science and Engineering
Level of Degree
Doctoral
Department Name
Optical Science and Engineering
First Committee Member (Chair)
Brueck, Steve
Second Committee Member
Hasselbeck, Michael
Third Committee Member
Malloy, Kevin
Document Type
Dissertation
Language
English
Recommended Citation
Seletskiy, Denis. "Ultrafast terahertz spectroscopy and control of collective modes in semiconductors." (2011). https://digitalrepository.unm.edu/ose_etds/40