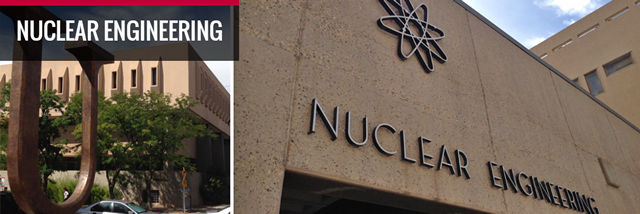
Nuclear Engineering ETDs
Publication Date
6-3-1968
Abstract
Cold traps have been used extensively for controlling oxygen concentration in sodium systems. Cold traps utilize the positive temperature dependence of the solubility of sodium monoxide in sodium and the resultant precipitation of sodium monoxide at low sodium temperatures. Sodium monoxide precipitation is here assumed to be heterogeneous because of the strong effect of surface area on the precipitation rate shown in previous packless cold trap experiments. Growth of crystals from a super-saturated solution is said to proceed according to the equation
where dm/dt is the rate of mass accumulation on the crystal, k is an over-all mass transfer coefficient including a diffusion step and a precipitation step, A is the crystal surface area, C is the bulk solute concentration and Ce is the equilibrium concentration at the temperature of the crystal. A packless cold trap of simple geometry and operating with a temperature gradient was used in this study. In the presence of the temperature gradient, the effective precipitation surface area was a function of the oxygen concentration in the sodium entering the cold trap. An equation describing the change in oxygen concentration with time was derived by performing a mass balance around the system using equation 1 and by accounting for the change in effective surface area with changing oxygen concentration. Eleven cold trapping runs were performed in which concentration was measured as a function of time. Tests were performed at various flow rates, initial oxygen concentrations and final oxygen concentrations. In general, the theoretical model fit the data quite well. Values of the mass transfer coefficients were calculated and were found to be a function of both temperature and flow rate. The dependence on sodium flow rate was proportional to Reynolds number to the 0.6 power. Variation of the mass transfer coefficient with flow rate implied that the precipitation process was diffusion controlled. An Arrhenius plot of the mass transfer coefficient indicated that the activation energy for the process was 3.0 kcal/mole which agrees well with the activation energy for diffusion of sodium monoxide in sodium reported as 2.4 kcal/mole. At 170° C and a Reynolds number of 1120, the mass transfer coefficient was 2.52 x 10-5 g O/cm2-h-ppm.Two dissolution test runs were performed in which sodium monoxide was dissolved from the cold trap into the sodium system. Oxygen concentration was measured as a function of time and mass transfer coefficients were calculated. At 200° C the mass transfer coefficient was 5.2 x 10-6 g 0/cm2 -h-ppm. No attempt was made to determine flow rate dependence. The activation energy for dissolution was 19.0 kcal/mole which is much higher than the activation energy for diffusion. The dissolution process was, therefore, dissolution rate controlled rather than diffusion controlled. The difference between the activation energy for dissolution and the activation energy for precipitation is equal to the heat of solution of sodium monoxide in sodium. The heat of solution determined from the solubility of sodium monoxide in sodium is 16.0 kcal/mole. Since the activation energy for dissolution was 19.0 kcal/mole, the activation energy for precipitation should be approximately 3.0 kcal/mole. This value would be applicable to precipitation rate controlled processes in the sodium monoxide-sodium system.
Document Type
Thesis
Language
English
Degree Name
Nuclear Engineering
Level of Degree
Masters
Department Name
Nuclear Engineering
First Committee Member (Chair)
Kenneth Edward Cox
Second Committee Member
Robert E. Cowan
Third Committee Member
Thomas T. Castonguay
Fourth Committee Member
Frank W. Clifford, Jr.
Recommended Citation
McPheeters, Charles Clellan. "Mass Transfer of Oxygen in Sodium Cold Traps." (1968). https://digitalrepository.unm.edu/ne_etds/123